A metallic alloy composed of chromium, cobalt, and nickel is the toughest material ever tested. This substance, known as CrCoNi, is highly resistant to breaking and has the potential to be used in the construction of aircraft or spacecraft. While it is also strong and malleable, its strength and ductility increase at lower temperatures, which is unusual for most other materials.
It is important to note that toughness and hardness are not the same things; toughness refers to a material's ability to resist fracturing, while hardness measures a material's resistance to deformation.
In addition to being highly malleable (a characteristic known as ductility in materials science) and resistant to permanent deformation (a measure of strength), this metal becomes even stronger and more flexible as it gets colder. This is a rare trait among materials, as most become less ductile and weaker at lower temperatures.
Toughness in Temperature
A research team from Lawrence Berkeley National Laboratory and Oak Ridge National Laboratory recently published a study in Science detailing their discovery of the toughest material ever tested. The material, an alloy of chromium, cobalt, and nickel, is both strong and malleable and becomes even more so at lower temperatures.
This is a rare trait among materials, as most become brittle and weaker at lower temperatures. According to Easo George, project co-lead and the Governor's Chair for Advanced Alloy Theory and Development at ORNL and the University of Tennessee, when designing structural materials, one may desire it to be substantial but also malleable and immune to fracture. Typically, it's a compromise between these properties. But this metallic alloy has both, and rather than becoming brittle during low humidity, it gets tougher.
CrCoNi is a type of high entropy alloy (HEA), which is a class of metals made up of equal proportions of each constituent element rather than a high proportion of one element with lower amounts of additional elements. This balanced atomic structure has been found to give some HEAs an unusually high combination of strength and ductility when subjected to stress, resulting in high toughness. HEAs have been the subject of much research since they were first developed around 20 years ago, but until recently, the technology to fully test their capabilities under extreme conditions was not available.
At temperatures near liquid helium (-424 Fahrenheit), the toughness of this material (measured in units of megapascals square root meters) is as high as 500. For comparison, the toughness of a piece of silicon is 1, the aluminum used in airplane frames is about 35, and some of the strongest steels have a toughness of around 100. According to research co-leader Robert Ritchie, a senior faculty scientist in Berkeley Lab's Materials Sciences Division and the Chua Professor of Engineering at UC Berkeley, 500, it's a staggering number.
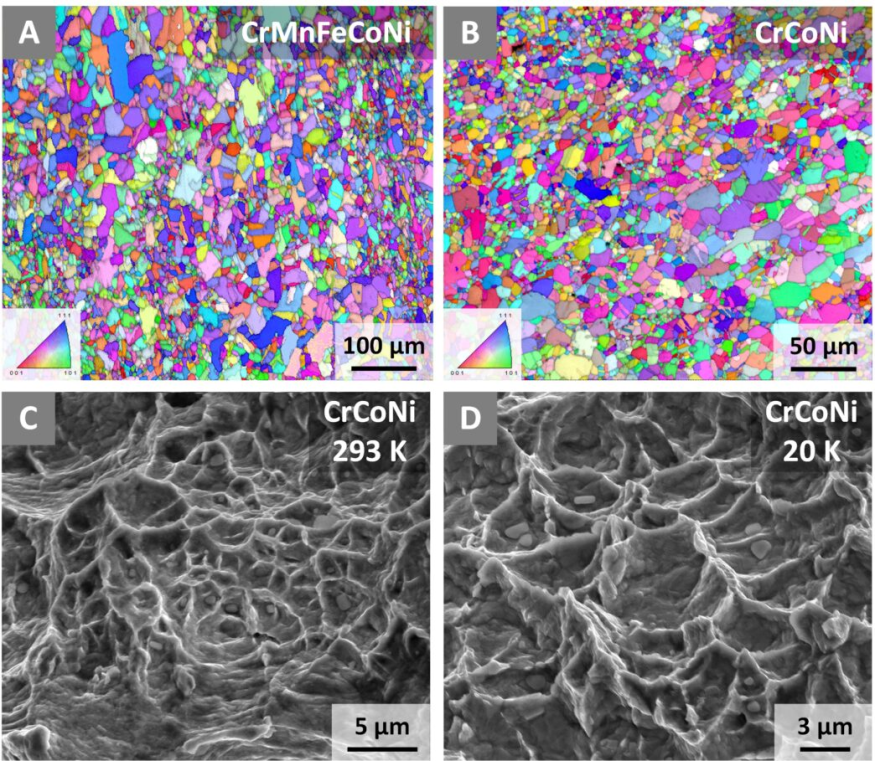
CrCoNi's Contender
Nearly a decade ago, Ritchie and George began experimenting with CrCoNi and another alloy called CrMnFeCoNi. When they cooled samples of these alloys to liquid nitrogen temperatures (-321 F) and subjected them to stress testing, they discovered impressive strength and toughness.
They were eager to follow up their work by testing the alloys at even colder temperatures, but it took them several years to locate facilities capable of stress testing samples in such a cold environment and to assemble a team with the necessary expertise and analytical tools to analyze the materials at an atomic level. However, their efforts paid off in the end, as the results of these tests proved to be worth the wait.
Numerous solid essences, including metals, live in a crystalline condition distinguished by a repeating 3D atomic routine called the unit cell. These unit cells make up a larger structure called a lattice, and the material's strength and toughness (or lack thereof) come from the physical properties of the lattice.
All crystals contain defects, such as dislocations, which are boundaries where an undeformed lattice meets a deformed lattice. When force is applied to the material, the shape change is accomplished by the movement of dislocations through the lattice. If the dislocations can move easily, the material is soft. If the movement of the dislocations is blocked by obstacles in the form of lattice irregularities, then more force is required to move the atoms within the dislocation, and the material becomes stronger. However, these obstacles also usually make the material more brittle, or prone to cracking.
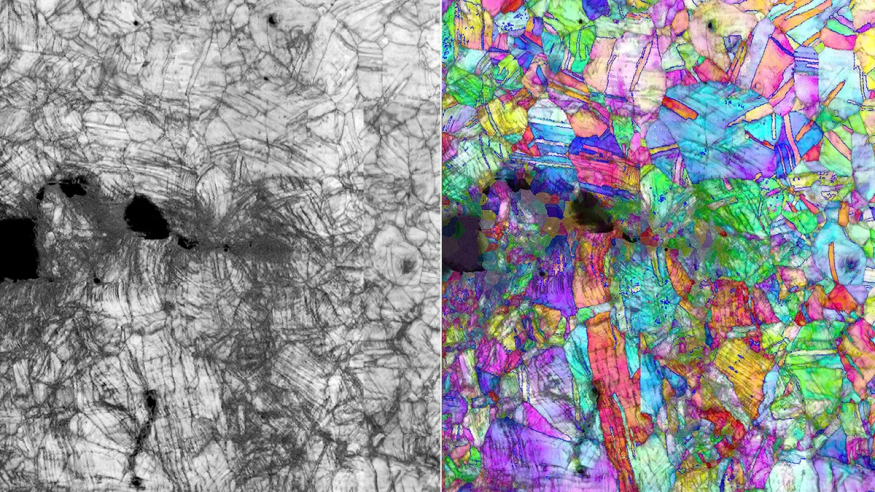
ALSO READ : Silkworm Silk Can Be Stronger Material, Compared to Spiders' Using Metal-Enriching Technology Study Claims
Relating to Crystal Structure
Ritchie, George, and their colleagues at various research institutions used neutron diffraction, electron backscatter diffraction, and transmission electron microscopy to examine the lattice structures of CrCoNi samples that had been fractured at room temperature and 20 K.
These techniques allowed them to generate images and atomic maps of the material. They found that the alloy's toughness is due to a combination of three types of dislocation obstacles that come into effect in a specific order when force is applied to the material. The first obstacle is created when moving dislocations cause areas of the crystal to slide away from other areas on parallel planes, resulting in a misalignment of unit cell patterns.
The second obstacle is called nanotwinned, which occurs when areas of the lattice form a mirrored symmetry with a boundary in between. Finally, if additional force is applied, the energy being put into the system causes the unit cells themselves to rearrange from a face-centered cubic crystal structure to a hexagonal close-packing structure. These obstacles block the movement of dislocations, making the material stronger, but also making it more brittle.
Combining New Products
As reported by NewScientist, the sequence of atomic interactions allows the metal to continue flowing while also meeting increasing resistance from obstacles, which helps it maintain its strength and ductility far beyond the point at which most materials would break under the same strain. This combination of mechanisms is what gives the material its exceptional properties.
These findings, along with other recent research on HEAs, may challenge long-held beliefs about the relationship between a material's physical characteristics and its performance. The structure of CrCoNi is relatively simple, consisting of grains, but when it is deformed, its structure becomes more complex and this transformation helps to explain its exceptional resistance to fracture. The CrMnFeCoNi alloy also performed well at 20 K but did not achieve the same level of toughness as the simpler CrCoNi alloy.
Now that the mechanisms behind the exceptional toughness of CrCoNi and other HEAs are better understood, these materials are one step closer to being used in special applications. While these materials are currently expensive to produce, they have the potential to be used in extreme environments where standard metallic alloys would be damaged, such as in the cold temperatures of deep space. George and his team at Oak Ridge are also exploring the possibility of creating alloys made of more abundant and cost-effective elements that could have similar properties, as there is currently a global shortage of cobalt and nickel due to their demand in the battery industry.
Check out more news and information on Technology and Innovation in Science Times.