Cosmologists have spent decades trying to figure out why the universe is so bland. Not only is it completely flat and smooth as far as the eye can see, but it's also growing at an ever-so-slowly rising rate even though naive calculations predict that space should have folded up by gravity and blown apart by repelling dark energy after the Big Bang.
To explain the flatness of the universe, physicists suggest a dramatic beginning to cosmic history: space swiftly swelled like a blimp at the commencement of the Big Bang, smoothing away any curvature. To explain the gradual expansion of space after the initial burst of inflation, some have proposed that our universe is merely one of many less hospitable universes.
Two physicists had already recently thrown prevailing wisdom about our vanilla cosmos on its head. Following the footsteps of Stephen Hawking as well as Gary Gibbons, the duo has produced a new calculation that suggests the simplicity of the universe is anticipated, rather than unusual. As per Neil Turok from the University of Edinburgh with Latham Boyle from the Perimeter Institute for Theoretical Physics at Waterloo, Canada, the universe is the way it is for the same reason that air distributes evenly around a room: Stranger choices are conceivable but very implausible. The cosmos may appear to be exceedingly fine-tuned and implausible, but [they're] saying, 'Wait a minute, it's the preferred one,' said Thomas Hertog, a cosmologist there at the Catholic University of Leuven in Belgium.
The Imaginary Paths
The intriguing conclusion is based on a mathematical technique that involves changing to a watch that ticks with mathematical formulas. Turok and Boyle were able to compute entropy, which looks to correlate to our universe, using the hypothetical clock, as Hawking demonstrated in the 1970s. However, the fictitious time trick is a circuitous approach to calculating volatility, and the meaning of the amount remains highly contested in the absence of a more rigorous technique. While physicists debate the analysis and prediction of the entropy calculations, many see it as a new landmark on the path to understanding the basic, quantum basis of space and time.
Turok and Boyle, regular collaborators, are known for developing novel and unconventional cosmological concepts. The year before, they used a technique established in the 1940s by physicist Richard Feynman to investigate how likely our universe is.
To describe the probabilistic nature of particles, Feynman envisaged that a particle would examine all potential pathways from start to finish: a perfect line, a bend, a loop, and so on. He invented a method for assigning a number to each path based on its likelihood and totaling the results. This "path integral" method evolved into a strong foundation for forecasting the behavior of any quantum system.
But as soon as Feynman made the path integral public, scientists saw a strange relationship with thermodynamics, the old study of temperatures and energy. Turok and Boyle's computation was made possible by this link between quantum theory as well as thermodynamics.
From a Physicist's View
Thermodynamics uses statistics to explain a system with many pieces, such as the billions of air molecules bouncing around a room, with only a few numbers. For example, temperature, simply the average pace of air molecules, provides an approximate estimate of the energy in space. Overall characteristics such as temperature and pressure characterize the room's "macrostate."
A macrostate, on the other hand, is a rudimentary explanation; air molecules may be organized in an infinite number of ways that all relate to one macrostate. If you move one oxygen atom to the left, the temperature does not change. A microstate is a unique microscopic configuration, and the amount of microstates that correspond to a specific macrostate defines its entropy.
Entropy provides physicists with a clear means of assessing the probabilities of various outcomes: the higher a macrostate's entropy, the further likely it is. There are more options for air molecules that organize themselves across the room than there are in a corner, for example. As a result, air molecules are expected to spread outside and remain spread out. The self-evident reality that likely outcomes are probable is becoming the famous second rule of thermodynamics: that perhaps the overall entropy of an isolated system tends to increase.
The connection to the route integral was undeniable: in thermodynamics, you sum up all conceivable system configurations. And the route integral adds up all conceivable pathways that a system can follow. There is one significant difference: thermodynamics works on probabilities, which seem to be positive numbers that simply add up. However, the number allocated to each path in the path integral is complex, implying that it contains the imaginary number I, the square root of 1. When complex numbers are combined, they can grow or shrink, capturing the wavelike character of subatomic particles, which can merge or cancel out.
However, scientists discovered that a simple transition may transport you from one domain to another. Make more time hypothetical (a motion described as a Wick revolution after the Italian scientist Gian Carlo Wick), as well as a secondary I enters the route integral, suffocating the first one and converting imaginary values to real probability. You can obtain a familiar thermodynamic equation by replacing that time variable well with the reverse of temperature.
This Wick technique led to Hawking and Gibbons' stunning discovery in 1977 at the end of a rapid sequence of theoretical discoveries concerning space and time.
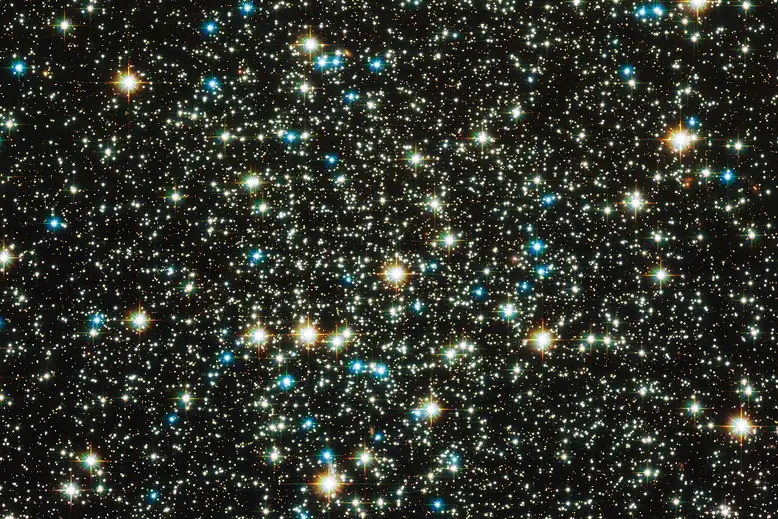
Scientific Concept of Space and Time
Decades before, Einstein's general theory of relativity demonstrated that time and space together constitute a cohesive fabric of the universe - space-time - and that gravity is just the propensity for things to follow the folds in space-time. Space-time can bend severely enough in extreme situations to form an impenetrable Alcatraz described as a black hole.
Jacob Bekenstein proposed the idea that black holes are flawed cosmic prisons in 1973. He reasoned that rather than eliminating the unpredictability of their meals and breaching the second rule of thermodynamics, the abyssal depths should absorb it. However, if black holes possess entropy, then they must also possess temperatures and emit heat.
Stephen Hawking, a skeptic, attempted to prove Bekenstein incorrect by doing extensive calculations as to how quantum particles act in the spherical shell of a black hole. In 1974, he discovered, to his amazement, that black holes can and do emit radiation. Further calculation supported Bekenstein's hypothesis: a black hole possesses entropy proportional to one-quarter the size of its singularity - the point at which an infalling item can no longer return.
Alternative Possible Universes
In the years thereafter, British scientists Gibbons as well as Malcolm Perry, then later Gibbons plus Hawking, have reached the same conclusion from a different angle. They put up a path analytic, which in theory adds up all the many ways space-time may bend to form a black hole. They then Wick-rotated the black hole, tracing the passage of time using imaginary numbers, and examined its form. They noticed that the black hole occasionally reverted to its starting condition in the fictitious time direction.
Immediately, Hawking as well as Gibbons Wick-rotated any of the most basic worlds possible - one having nothing other than the dark energy embedded into space itself. The empty, expanding universe, known as a "de Sitter" space-time, features a horizon beyond which space grows so rapidly that neither signal from there would ever reach a spectator in the center. Gibbons and Hawking determined in 1977 that, like a black hole, a de Sitter universe has an entropy equal to one-fourth the size of its horizon. Once again, space-time appeared to contain a finite number of microstates.
They obtained a more adaptable equation for cosmic entropy by Wick-rotating the roller-coaster's longest from a more realistic class of universes. The formula returns the number of matching microstates given a large spectrum of cosmic macrostates determined by radiation, matter, curvature, and a dark energy density (much like a range of temperatures as well as pressures defines multiple conceivable environments of a room). Turok and Boyle made their findings public in early October.
RELATED ARTICLE : The Universe is Basically Left-Handed: Here are Some Reasons Why
Check out more news and information on Universe on Science Times.