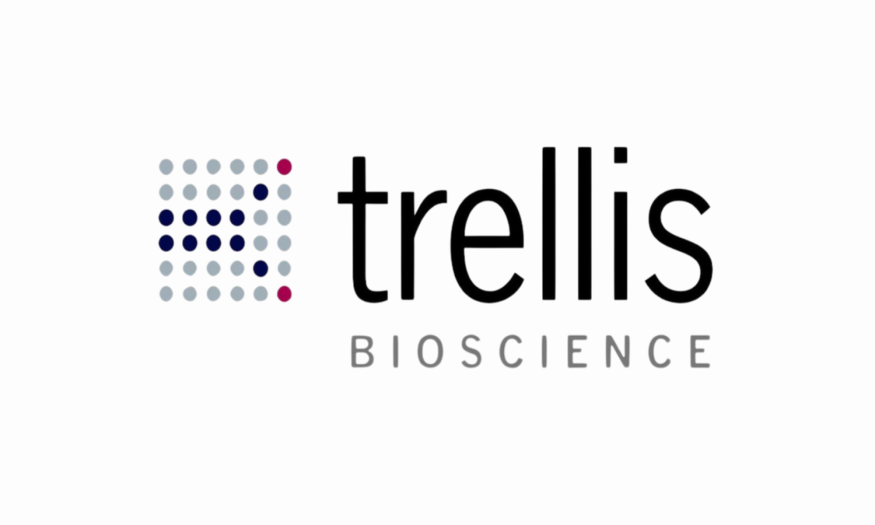
In the natural world, biofilm is a complex aggregate of bacteria and a bacterially produced extracellular matrix that provides a protective environment for the microorganisms, shielding them from natural antibiotics and acute environmental stressors such as nutrient restriction, desiccation, UV radiation, extreme temperature, and pressure, and high alkalinity or acidity. Biofilms affect industrial pipes and underwater structures and are ubiquitous in soil [1].
In the human body, bacteria in biofilms are protected from attack by pharmaceutical antibiotics and by the immune system, allowing persistence even in hostile conditions. Within the biofilm matrix, bacterial cells adopt a defensive physiological state that includes coordinated expression of multiple resistance genes [2].
Traditional antibiotics are becoming increasingly ineffective against a growing number of bacterial species due to mutations in specific genes [18]. This classical form of antibiotic resistance can often be overcome by an increased dose of the antibiotic. The resistance due to biofilm, by contrast, cannot generally be overcome by increasing the dose. Bacteria in the biofilm-associated "sessile" physiological state are typically resistant to antibiotic concentrations up to 1,000 times higher than that of their "planktonic" (free-growing) counterparts, posing a significant challenge for treatment. Moreover, the biofilm environment facilitates the development of classical resistance through the exchange of DNA fragments among bacteria ("resistance transfer vectors") [3]. Such transfers can occur between different species, facilitated by biofilms that are often polymicrobial [4]. Biofilm is a dynamic environment that includes the shedding of bacteria that convert to the planktonic physiological state, which can contribute to recurrent infections.
Appreciation of the importance of biofilm has been growing over the past three decades. Early attempts to counteract biofilm focused on the signaling system that regulates the associated shift in the physiological state ("quorum sensing"). However, due to the complexity and pathway redundancy of the process, drugs of this sort have not been very successful in combating infections, with none approved to date [5]. Trellis Bioscience's TRL1068 is the first clinical-stage agent for a new class of drugs called biofilm disruptors [6]. Clinical proof-of-concept for TRL1068 was achieved in patients with periprosthetic joint infections (PJI) [7]. Lower extremity (knee and hip) total joint arthroplasties are among the most commonly performed surgical procedures in the USA, providing a substantially improved quality of life for most patients. However, despite advances in perioperative antiseptic protocols, surgical technique, and operating room sterility, infection remains the most common cause of prosthetic joint failure within the first year after primary arthroplasty [8], affecting ~2% of patients.
The full prevalence of biofilms in human disease is likely underestimated as there are no widely accepted biomarkers. Nonetheless, biofilms are known to be present in a wide range of human tissues and are associated with a substantial fraction of all disease conditions for which antibiotics fail to eliminate the infection and associated inflammation [9]. For example, bacterial biofilm predominates in both acute and chronic lung infections [10], broadening the potential utility of TRL1068 to include a variety of bronchial and bloodstream infections. This mechanism for antibiotic resistance is of particular concern for hospital-acquired infections, which pose a high risk for immunocompromised patients. Approximately 50% of biofilm-associated infections occur in patients with indwelling medical devices such as catheters, cardiac pacemakers, joint prostheses, and prosthetic heart valves. These surfaces provide favorable topography for bacterial attachment and biofilm formation. Antibiotics like imipenem and colistin can reduce but not eliminate biofilms; their significant toxicity and side effects make it challenging to achieve effective in vivo concentrations [2]. Moreover, intermittent use of antibiotics against biofilm-associated bacteria promotes the development of classical drug resistance [17].
None of the commercially available antibiotics were discovered using sessile bacteria but rather were based on the much easier assay of killing planktonic bacteria [11]. The resulting treatments have worked very well for nearly a century. However, such antibiotics have only limited efficacy against biofilm-embedded bacteria, contributing to today's clinically significant antimicrobial resistance (AMR). Despite the growing awareness of biofilm in the research community, the medical and pharmaceutical communities still perceive AMR as being primarily due to the classical mechanism of mutated genes. This incorrect perception is based on the lack of effective drugs to overcome antibiotic resistance due to biofilm.
The mechanism of action of the new class of biofilm disruptor drugs is to change the bacterial environment, making bacteria susceptible to the traditional antibiotics that work well to eliminate planktonic bacteria. By eliminating biofilm, this new class of drugs can reduce the hospitable environment for gene transfer, thereby decreasing classical resistance and restoring the effectiveness of traditional antibiotics. Unlike the strategy of interfering with quorum sensing, which is variable across different bacterial species, biofilm disruptors are focused on the structural components shared by diverse bacteria to enable the formation of polymicrobial biofilms [12].
As is often the case for a new drug class, it is important to choose an initial indication that provides a clear demonstration of the novel mechanism. Chronic PJI (periprosthetic joint infection) is ideally suited to provide that demonstration. The current standard of care involves a burdensome two-stage replacement surgery protocol. After removal of the infected prosthesis, an antibiotic-eluting spacer is put in place for 6–8 weeks, followed by a second surgery to implant a fresh prosthetic joint. Measuring the adherent bacterial burden on the initially explanted prosthesis is an established method for characterizing the infection in order to select the most effective antibiotics [13]. In the recently completed clinical trial of TRL1068 [7], that bacterial burden was shown to be markedly reduced compared to historical experience, including complete eradication in several cases. This result is consistent with a published mouse model for which biofilm disruption was demonstrated using direct visualization of the implant surface by scanning electron microscopy [6]. In this Phase 1 proof-of-concept study, only single doses of TRL1068 were used (at escalating levels). Although antibodies have a half-life in the bloodstream of ~18 days, further studies will use multiple doses to eradicate chronic PJI infections without the need for prosthesis replacement. For patients hospitalized in the intensive care unit, TRL1068 has the potential utility to treat acute infections that are often associated with biofilm formation while also preventing the development of new hospital-acquired infections.
The standard of care replacement surgery for chronic PJI is associated with a mortality rate five times higher than aseptic replacements within the first year, adding over $3 billion annually to US healthcare costs (direct costs, with indirect costs more than double this figure due to the lengthy immobility and substantial recurrence associated with this regimen) [14]. TRL1068 presents a more efficient treatment option without the burdensome surgeries, potentially reducing expenses for healthcare systems and insurers, enhancing patient outcomes, and lowering recurrence of the joint infection, which currently affects up to a quarter of patients treated for PJI.
Monoclonal antibodies are the fastest-growing segment of the pharmaceutical industry, with revenues in 2023 exceeding $230 billion worldwide and projected to more than double over the next ten years [15]. Applying this proven technology to infectious disease targets has lagged behind its use for cancer and inflammatory diseases [16]. Pathogens, with their high mutation rates, were perceived as particularly hard to attack, but work over the past ten years has established that many pathogens have functionally critical components that cannot mutate without losing viability.
TRL1068 is a monoclonal antibody that targets the DNABII family of bacterial proteins that provide an important scaffolding function for holding the biofilm matrix together. Removing that scaffolding protein leads to the biofilm breaking down over the course of several hours, as measured in vitro [12]. It is difficult for bacteria to escape this mode of pharmaceutical intervention, in part because the binding site for TRL1068 is functionally necessary. In addition, most of the biofilm matrix is built up from the contents of dead bacteria; accordingly, since the target bacterial protein is only exposed to the antibody after the producing bacterial cell is dead, there is no growth advantage to an escape mutation. This feature is important for enabling the widespread use of TRL1068 as a front-line therapy, which stands in sharp contrast to newly approved antibiotics, whose use is often restricted in order to reduce the development of antibiotic resistance.
TRL1068 binds tightly to a site on the DNABII proteins found in most clinically significant bacteria, including both Gram-positive and Gram-negative species. The high conservation of this site is a hallmark of a functionally critical component. Tight binding is important to prevent the DNABII proteins from being released back at the site of infection or elsewhere in the body [19], which could contribute to the formation of biofilm-protected reservoirs of bacteria. Another favorable feature of TRL1068 is that its genes were cloned directly from human antibody-producing white blood cells. Such native human monoclonal antibodies have a low risk of off-target reactivity that can contribute to toxic side effects and to the development of anti-drug antibodies; they are, therefore, more suitable for repeat dosing than antibodies from other sources.
While AMR cannot be resolved with one new magic antibiotic, the new mechanism of action of biofilm-disrupting drugs has the potential to make a broad range of established antibiotics more effective against biofilm-embedded bacterial pathogens, thus extending the major success of the past century of medical progress in controlling infection.
To learn more, visit trellisbio.com.
References
[1] Yin W, Wang Y, et al. Biofilms: The Microbial "Protective Clothing" in Extreme Environments. Int J Mol Sci. 20(14):3423 (2019).
[2] Roy R, Tiwari M, et al. Strategies for combating bacterial biofilms: A focus on anti-biofilm agents and their mechanisms of action. Virulence 9(1):522-554 (2018).
[3] Metzger GA, Ridenhour BJ, et al. Biofilms preserve the transmissibility of a multi-drug resistance plasmid. npj Biofilms Microbiomes 8:95 (2022).
[4] Stevenson P, Marguet M, Regulski M. Biofilm and Hospital-Acquired Infections in Older Adults. Crit Care Nurs Clin North Am. 35(4):375-391 (2023).
[5] Bhattacharya SP, Karmakar S, et al. Quorum sensing inhibition and antibiofilm action of triterpenoids: An updated insight. Fitoterapia 167:105508 (2023).
[6] Burke ZDC, Hart CM, et al. Monoclonal Antibody Disrupts Biofilm Structure and Restores Antibiotic Susceptibility in an Orthopedic Implant Infection Model. Antibiotics (Basel) 12(10):1490 (2023).
[7] Conway J, Delanois RE, et al. Phase 1 study of the pharmacokinetics and clinical proof-of-concept activity of a biofilm-disrupting human monoclonal antibody in patients with chronic prosthetic joint infection of the knee or hip. Antimicrob Agents Chemother. Jul 16:e0065524 (2024) Epub ahead of print.
[8] Mathis DT, Hirschmann MT. Why do knees after total knee arthroplasty fail in different parts of the world? J Orthop 23:52-59 (2021).
[9] Perry EK, Tan MW. Bacterial biofilms in the human body: prevalence and impacts on health and disease. Front Cell Infect Microbiol.13:1237164 (2023).
[10] Koplen M, Kragh KN, et al. Bacterial biofilms predominate in both acute and chronic human lung infections. Thorax 77:1015-1022 (2022).
[11] Coenye T, Bové M, Bjarnsholt T. Biofilm antimicrobial susceptibility through an experimental evolutionary lens. npj Biofilms Microbiomes 8:82 (2022).
[12] Estellés A, Woischnig AK, et al. A High-Affinity Native Human Antibody Disrupts Biofilm from Staphylococcus aureus Bacteria and Potentiates Antibiotic Efficacy in a Mouse Implant Infection Model. Antimicrob Agents Chemother. 60(4):2292-301 (2016).
[13] Trampuz A, Piper KE, et al. Sonication of removed hip and knee prostheses for diagnosis of infection. N Engl J Med. 16;357(7):654-63 (2007).
[14] Parisi TJ, Konopka JF, Bedair HS. What is the Long-term Economic Societal Effect of Periprosthetic Infections After THA? A Markov Analysis. Clin Orthop Relat Res. 475(7):1891-1900 (2017).
[15] Vision Research Reports (2023). Monoclonal Antibodies Market – Global Industry Analysis, Size, Share, Growth, Trends, Revenue, Regional Outlook and Forecast 2024–2033.
[16] Marston HD, Paules CI, Fauci AS. Monoclonal Antibodies for Emerging Infectious Diseases – Borrowing from History. N Engl J Med. 378(16):1469-1472 (2018).
[17] Usui M, Yoshii Y, et al. Intermittent antibiotic treatment of bacterial biofilms favors the rapid evolution of resistance. Commun Biol. 6:275 (2023).
[18] Mancuso G, Midiri A, Gerace E, Biondo C. Bacterial Antibiotic Resistance: The Most Critical Pathogens. Pathogens 10(10):1310 (2021).
[19] Devanaboyina SC, Lynch SM, Ober RJ, Ram S, Kim D, Puig-Canto A, Breen S, Kasturirangan S, Fowler S, Peng L, Zhong H, Jermutus L, Wu H, Webster C, Ward ES, Gao C. The effect of pH dependence of antibody-antigen interactions on subcellular trafficking dynamics. MAbs 5(6):851-9 (2013).